RESEARCH INTERESTS
Emergent properties can be a versatile viewpoint to understand how living systems acquire complex structures and functions. We believe that, to illuminate this point of view and elucidate the unrecognized mechanisms underlying their morphogenesis and functions, it is the key to bridge the developing theory methodologies and concepts for mechanics and statistical physics to biological systems. However, there is still a big gap between such dynamic phenomena observed in real living systems and recently accumulated knowledge in the physics field. Under this circumstance, we are seeking for the possible mechanisms behind dynamic emergent phenomena in living systems, from theoretical biophysicist views.
​
Here, for more details, we list up our studies on this motivation.
Mechanical robustness/plasticity of phase-separation structures in a chromatin (SD, RD, TH)
Organization of a chromatin inside a nucleus regulates the gene expression of that cell and determines its specific functionality. A typical nuclear landscape appear to be phase separated into transcriptionally active euchromatic and repressive heterochromatic regions. While the chromatin retains an open structure in the euchromatic region, it is highly dense in the heterochromatic region
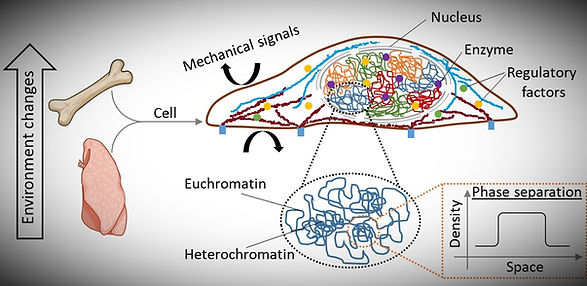
such that the transcription factors cannot access genes coded in that region, and consequently those genes remains untranscribed. Recent investigations has reveled that mechanical environment of a cell has significant effect on this phase separated landscape of the nucleus, viz., on the total content of the heterochromatic segments and their morphology. Moreover, the presence of several chemical agents within the nucleus plays important roles stabilizing those heterochromatic structures, and thereby determining cell functionality. Rakesh is investigating several length and time scales of the chromatin structure induced by various chemo-mecahnical signals from a theoretical physicist's point of view.
Tissue homeostasis with cell-cell and cell-ECM mechanical interaction (YL and TH)
​

Cellular events are orchestrated for the maintenance of tissue homeostasis which is crucial for human beings' health and life. Mechanical forces generated from actin-myosin network play pivotal roles in controlling cell shape, intercellular adhesions and their communication with extracellular matrix(ECM). Yuting is interested in how multicellular mechanics are involved in determining the homeostatic events such as cell division, cell death and cell cycle progression\arrest by affecting the mechanical dynamics of tissues and ECM.
​
Theoretical models such as Vertex/Voronoi model, self-propelled models, contiuum model for viscoelastic material are extensively used to reproduce complex phenomena observed in tissue experiments in vitro. ​
Current projects of Yuting:
-
A novel long-range force propagation with sharp deformation peak in ECM induced by cell-apoptosis (collaborated with Toyama Yusuke, paper in preparation);
-
Aggregation of cells in S-G2-M phase as a result of heterogeneous tension distribution in tissue and its impact on the synchronization of cell cycle progression;
-
The emergence of rare topology "skewtoid" (apical-basal T1 transitions) during the embryotic development of Drosophila, collaborated with Jean-Franqcois at CNRS (France) and Timothy Saunders at University Warwick (UK) .
Dynamic multicellular assemblies of migrating cells (TH. GMG joined.)
The emergence of coherent dynamics is a key aspect for understanding mechanisms underlying morphogenesis and functional processes of living systems. Even the fundamental unit of living systems – cells – can form many types of multicellular assemblies with coherent dynamics, which is called dynamic self-organization (DSO). This series of works focus on such DSO exhibited by cells migrating around on a substrate. Recently, several studies have suggested that intercellular methods of contact communication, such as contact following (CF) and contact inhibition/attraction of locomotion (CIL/CAL), play crucial roles for such DSO of migrating cells.
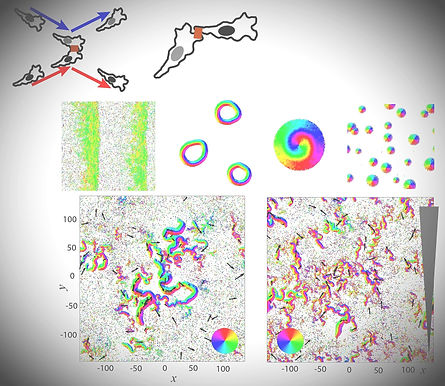
Tetsuya proposed and numerically investigated a theoretical model of DSO of migrating cells caused through various types of contact communication. This proposed model can reproduce experimentally observed DSO only just by tuning the strengths of each types of contact communication. The model further revealed a novel form of collective migration with highly dynamic structures, termed snake-like dynamic assembly (bottom subfigures in the right figure). These findings may open up new ways to understand the generation mechanisms and selection principles underlying emergence of dynamic multicellular organization. (TH, Phys. Rev. E 2019; Phys. Rev. Lett. 2020.)
Using the same model framework, TH succeeded to reproduce computationally the traveling density wave dynamics of a mutant Dictyostelium cells (social amoebae), which was discovered by his experimental collaborators in RIKEN and Tsukuba University. (Hayakawa, TH, ..., Shibata, eLife 2020)
Dynamics and mechanics of a cortical cytoskeleton (TH)
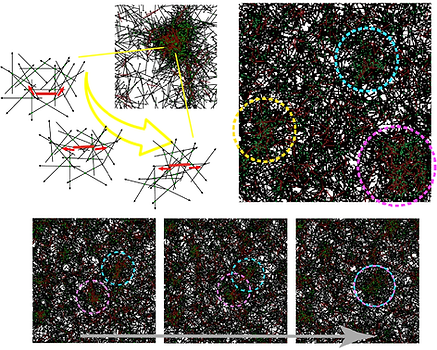
Cytoskeleton is, from the mechanistic perspective, regarded as the gel composed of semi-rigid polymer chains containing molecular motors. Structure and static mechanical feature of a cell cortical cystoskeleton, which consists of actin filaments, myosin molecular motors and crosslinker proteins, has been well investigated, but there are a lot of unexplored things left on its dynamics. Tetsuya is investigating the dynamics and motor-induced stress in such a cell cortical cytoskeleton. With his collaborator, he established and analyzed a mathematical model to calculate the dynamics of and evaluate the contractile stress in a fluidic actin myosin cytoskeleton, in which cytoskeletal elements can move around and undergoing turnover (TH and G. Salbreux PRL 2016).
​
Recently Tetsuya applied this theory to explain the mechanism of periodic propagation of density waves seen in the reconstituted cortex in a micron-meter scale droplet. This phenomenon was found by experimental collaborators in Kyushu, Waseda and Kyoto Universities. They together revealed its role for positioning of a structure in the micro environment. (Sakamoto, Tanabe, TH, ...., Maeda, Miyazaki, Nat Commun 2020)
Mechanism of autonomous unidirectional cell movement in an epithelial tissue (TH)
​
Bodies of multicellular organisms are covered by epithelial tissue, in which cells are tightly packed in columnar shapes and from the top view cells look like the polygons. Tetsuya applied a mathematical model called a 2D cellular vertex model based on the mechanics at the apical junctions and actomyosin belt, to explore the mechanism of collective cell movement of an epithelial tissue found by the collaborators from the developmental biology field in RIKEN CDB and Tohoku University; unidirectional rotation of the disc-shaped epithelial organ by the epithelial tissue-autonomous mechanism, observed during morphogenetic process of a male fruit fly.

Tetsuya together with the collaborators elucidated the novel mechanism of such autonomous unidirectional motion of an epithelial tissue. (Sato, TH, Maekawa, ..., Shibata, Kuranaga, Nat Commun 2015; TH et al. Front. Dev. Cell Biol 2017). He is also collaborating with the theorists in RIKEN BDR to explore other possible mechanisms of epithelial tissue motion (Yamamoto, TH and Shibata, Phys. Rev. Research 2020). Meanwhile, he is now trying to integrate the theoretical models of such apical-driven epithelial dynamics and collective migration on a substrate (mentioned above) to seek for the new form of cooperated behaviors in an epithelial tissue.
For more about Tetsuya Hiraiwa's works, visit his personal website.
​
For more about Yuting Lou's works, visit her Researchgate page
​
For more about Rakesh Das's works, visit his personal website.